
X-ray photoelectron spectroscopy (XPS) is the term used to describe photoelectron spectroscopy that uses excitation energies greater than 100 eV. Typical excitation energies are 1486.6 eV (characteristic X-ray line of aluminum, referred to as Al Kα ) or 1253.6 eV (Mg Kα line).
Due to the high excitation energy, XPS mainly extracts electrons from energetically lower shells, i.e. electrons from trunk levels are observed. This is why XPS is often referred to as "core level spectroscopy", in contrast to UPS, where the excitation energies are below 100 eV and electrons from the valence band are observed.
All photoelectron spectroscopy is based on the photoelectric effect discovered by Einstein and described in 1905. This means that electrons are released by the irradiation of energy in the form of light, for example. Furthermore, these electrons have a defined kinetic energy, which is related to their binding energy and work function. This is illustrated by equation 1.1.
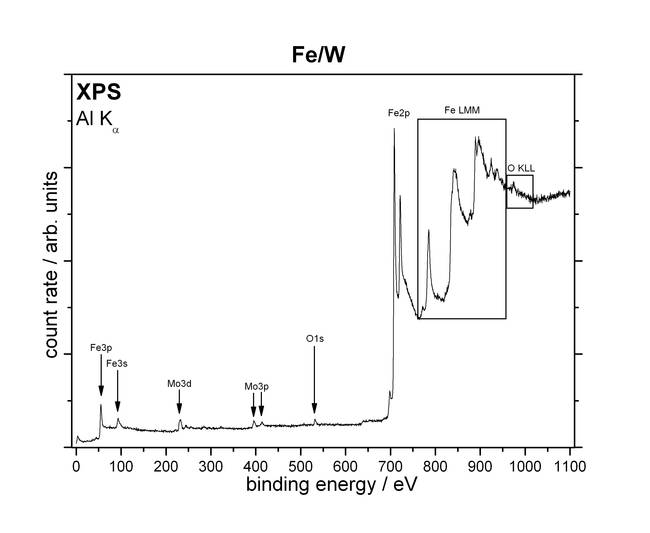
An energy-dispersive element can be used to detect the emitted electrons as a function of their kinetic energy.
A further process, the Auger process, takes place in parallel to the photoelectrons triggered by the irradiated X-ray light. In this process, electrons fall from a higher shell into the hole left by the photoelectron. The excess energy is transferred to another electron, which can then leave the atom (see Auger electron spectroscopy).
As the binding energy of the photoelectrons depends on the orbital from which they were released and is therefore also element-specific, XPS can be used to carry out chemical analyses of surfaces. The depth information is higher than with UPS (lower excitation energy) or AES (greater scattering losses inside the solid) and is around 10 nm.
A qualitative and quantitative chemical analysis of a surface is possible with XPS, and statements can also be made about chemical bonds on the surface. By shifting the binding energy of the electrons of a certain orbital (e.g. O 1s), the chemical environment of the electrons of this orbital can be determined.
For example, oxygen atoms can be bound to carbon or hydrogen atoms, whereby the binding energy shifts specifically depending on the type of molecule formed (e.g.CO2, CO, OH, H2O). This is also known as a "chemical shift". One example is the determination of the oxidation states of iron. This manifests itself in a chemical shift of the Fe 2p orbital. For a pure Fe2O3, for example, the signal of the Fe 2p 3/2 orbital shifts by approx. 4 eV to a higher binding energy. For a pure FeO, the signal only shifts by approx. 3 eV. This means that the III. oxidation state and the II. oxidation state differ by 1 eV.
For further information see here.